These Wings Were Made for Running: An Interview with Kenneth Dial
Flight as a case study in the evolution of complex anatomical structures
Jay Worthington and Kenneth Dial
The question of how flight evolved has fascinated biologists (and the general public) since Charles Darwin published The Origin of Species in 1859. Did it evolve from gliders who launched themselves from trees and developed the ability to flap their wings and fly (the arboreal model)? Or did it evolve from ground-based animals who developed wings which could carry them into the sky (the cursorial model)? After almost a century-and-a-half of study, the question still has not been definitively answered. A recent experiment by Kenneth Dial, Professor of Biology and Director of the Flight Laboratory at the University of Montana, investigated Wing-Assisted Incline Running (WAIR) and the uses to which modern birds can put partially developed wings. Jay Worthington spoke with him by phone.
Cabinet: Can you describe the mechanics of Wing-Assisted Incline Running?
Kenneth Dial: WAIR is a mechanism by which birds use their forelimbs to help the hind limbs enhance locomotor performance by improving traction. The easiest analogy to use is car spoilers. Some race cars use a mobile wing spoiler in the back. The spoiler is in a neutral position as the car travels down a straightaway. But as the car comes into a turn, it has the tendency to spin out, and so the spoiler engages and provides a downward force to improve traction on the rear tires. With birds, this process seems nonintuitive to observers, but once you get a sense of what the animal is doing with its wings, in slow motion, it’s like Homer Simpson going “D’oh!” However, initially it’s nonintuitive because we think of wings as taking animals to the sky. We normally don’t think of wings as hugging animals closer to the ground.
So the ability to use its wings to control traction can give an animal a competitive advantage by giving it the ability to, say, run up trees?
Any improvement in an animal’s ability to escape predators by taking advantage of vertical terrain features, whether they are little 45-degree inclines or vertical faces, increases the likelihood that it will survive and reproduce, and that’s the punch line.
Could you quickly describe the research that you used to test the WAIR theory? How were the experiments designed?
I’ve been interested in the evolutionary origins of flight for a long time, and the lack of direct data in the field is frustrating. There’s a limit to what we can learn from fossils, and so I’d been looking for a research project that could shed more direct light on the earliest flyers. I decided to focus on galliform birds like the chukar partridges in the lab films you’ve seen, because they, like their dinosaur predecessors, are ground animals, bipedal runners, and they have an unusual development relative to most modern birds. Most birds grow up in a nest, confined and immobile until they’re ready to fly. But these birds are born on the ground and have to rapidly develop effective locomotion or they’re somebody’s dinner. They have to become good runners right out of the egg, before they have fully developed wings and flying abilities, and so they seemed like they could provide an appropriate subject through which to examine the possible utility of the sort of partially developed flight structures which we see early in the fossil record.
So we started to do some basic studies with these birds. I was interested in quantifying how their wings worked during the first 60 days of life. I wanted to measure how they used their proto-wings, looking at how they developed and at how far and how well the young chicks could fly each day. My teenage son, Terry, helped me with the initial experiments, and we developed some tests by which we could push the birds to the maximum of their vertical and horizontal flight abilities each day. We would separate the chicks from their nest, a box which we would elevate out of reach, and the birds’ efforts to return to their siblings allowed us to measure their developing flying abilities. After a short while, we decided to more accurately recreate the birds natural living conditions, and we elevated their nests on bales of hay. For these new experiments, I recruited the efforts of my son and his friend, Ross Randall, to assist me in collecting data. Immediately after we made that change in the experiment, I was away for a week and left the measurements in my son’s hands. When I came back, I asked him how the research was going, and he told me that the birds were cheating, that they weren’t flying back up to their elevated nests but were running up the vertical sides of the bales of hay. That was the beginning of our epiphany—we set up high-speed video to precisely record what was happening, and we saw quite clearly that these birds were using their wings in a novel, previously unobserved way, to run rather than fly over vertical obstacles. We then set up a series of new experiments to more precisely measure the phenomenon, and it was that research which led to the WAIR theory.
Nobody had ever studied this before?
Nobody had. But the reason this hasn’t been found before is that it happens in very brief, explosive spurts of activity, such as when the birds are escaping predation or they’re going to a roost. They usually want to be alone when they do that, so it wouldn’t be something that you’d see very often, except under controlled conditions. It also requires very high-speed video in order to effectively study, because these animals will move twenty feet up a tree in less than a second. You just see a flutter, and it looks like an animal going through convulsions. But when you shoot it with high-speed film, it looks like ballet, like Baryshnikov.
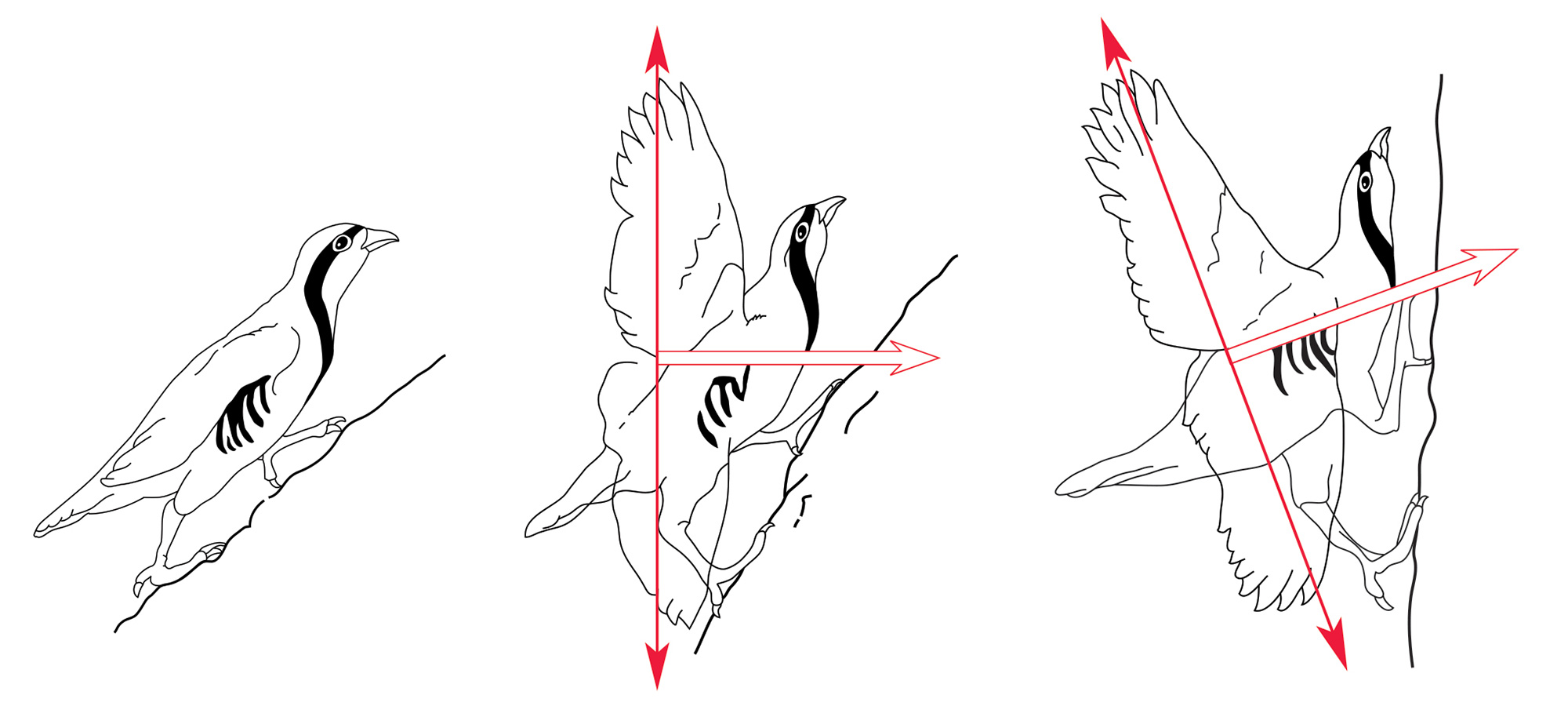
In addition to juvenile birds, you also took films of adult birds in your experiments. How did you get them to cooperate? How did you get them to run and not fly? Presumably the adults were fully capable of flight.
It’s true that the adult birds in our experiments can fly, but in our tests they preferred to run approximately 95 percent of the time. The birds’ internal muscle anatomy probably provides the best explanation for that. As most people probably first learned at the Thanksgiving dinner table, a ground bird’s breast muscles are composed of white meat, and its thighs and legs are composed of dark meat. The thigh and leg muscles are darker because they are largely aerobic, and aerobic muscle is densely provided with blood vessels and is infused with blood. White muscle, on the other hand, is anaerobic. It has very high power output, but like all anaerobic muscle it fatigues extremely rapidly. In general, an animal would prefer to rely on aerobic, rather than fast-fatiguing anaerobic, muscles, and so it makes sense that ground running birds show a preference for running rather than flying, when either method would provide adequate means for overcoming a vertical obstacle.
Does that muscular distribution tend to distinguish strong flyers from weak flyers?
That isn’t a simple question to answer, but, in general, aerobic muscle in the wings is one of the characteristics of good flyers. Power-to-mass ratio is also extremely important, and thus most birds are relatively small. Being small also allows you to have better acceleration and turning, and so smaller birds tend to have better flight performance and to be better able to evade predation. Predation seems to be a universal selective pressure on animals, and on birds in particular, that helps explains many of the evolutionary trajectories we observe. I would argue that the origins of flight and the characteristics of flyers that we’ve been discussing all make the most sense when we start to think about them as the results of predation pressures on birds.
What evolutionary steps distinguish ground birds from more specialized flyers?
Well, the strongest flyers only appeared once parental care became sophisticated enough to build a refuge that the birds could stay sequestered in, and once the parents, in addition to building a complex nest in an elevated site, would also feed and actively protect the vulnerable kids. The chicks could then race developmentally to become adult size and to launch out of the nest as fully developed flyers. In other words, you don’t see little baby eagles flying around, or bee-sized hummingbirds flying around, and that’s because they grow to the adult size before they leave the nest.
So they don’t need to develop intermediate structures.
That is correct. And they can start to jettison this hind limb luggage and become even better flyers. So the very best flyers are those that have great parental care, are raised in a very secluded, predator-free nest, and have almost abandoned their legs. Some of them, like swifts, swallows, and tropical birds, can’t even walk. Other than for perching, they don’t need legs at all, and they’ve invested all of their muscle mass into their forelimbs, which makes them extraordinary flyers.
In the fossil record, what’s the best estimate of the gap between the appearance of ground-based running birds with less-developed flight abilities and full-blown flyers that have abandoned the ability to walk and run?
It’s only recently that fossil discoveries have been made which could start to answer that question. Caudipteryx, for example, is a fossil with long hind legs and only partially developed wings, though it appears that its wings probably would have been adequate to provide utility through the process of wing-assisted running.
Has flying generally been a popular evolutionary choice?
Well, there is a kind of evolutionary inertia at work here, what some people might call a constraint. An animal inherits a commitment to a body plan, whether it’s a mammal or a bird or a reptile, and it isn’t easy to change such a commitment over the course of evolutionary change. But of the 13,000 species of warm-blooded animals, which include birds and mammals, 10,000 of them fly. It’s also important to note that while flying is expensive per unit of time—much more so than either swimming and walking—it nevertheless is the most efficient form of locomotion per unit distance traveled, and thus it provides evolutionary advantages in itself as an adaptive response to selective pressures.
So it has been a very popular strategy.
Yes. And of all the animals on earth, of which insects, with over a million species, are the largest group, by far most animals fly. Flying is not a necessity for success, of course. Chipmunks or squirrels, with their highly evolved abilities to move in three dimensions, are highly functional. If an animal has sufficient resources to live on within its cruising range, then it won’t face much pressure to develop flight. Examples—flightless cormorants or parrots come to mind—of birds which have abandoned flight when they escape predation, by becoming isolated on an island, for instance. It seems likely that predation may be more tied to the origin and utility of flight than just its potential to make available an increased range of resources.
So remove predation and the expense of flight stops looking worthwhile.
Exactly, as long as there’s food available within cruising range. Rails and the Galapagos Cormorant are starting to go that way. As did the Dodo bird and many other species that have since become extinct.
Which gets us back to the evolutionary questions that motivated your research.
The main point I really want to make clear in describing this project is that I was very interested in analyzing the utility that a partially developed wing could provide to an animal. The problem of describing the development of intermediate structures has been one of the biggest issues in the debate over the evolution of flight and in the debates over the evolution of complex structures generally. How does an animal develop from having no wing, or from having only the most rudimentary of wing-like buds, to having a fully functional wing? What are the evolutionary transitions, the intermediate plateaus, from which animals would derive benefit until they had developed full-fledged wings and flying abilities?
So that the evolutionary explanation wouldn’t have to depend upon quantum jumps.
Absolutely. Describing possible intermediate structures is, it seems to me, the key to the evolutionary discussion. It simply isn’t satisfying to assume that dramatic evolutionary leaps have occurred. That’s why the arboreal theory of the evolution of flight has been so powerful to many ornithologists. There are many examples of gliders today, and it seems very intuitive that all a glider would have to do is to start beating its wings as a first step along the path to flight. That seems very sensible.
It implies utility in the intermediate evolutionary stage.
That’s right. But there is one major problem with that approach. There are hundreds, if not thousands, of non-avian gliders and parachuters—everything from frogs which jump out of trees, to flying snakes, lizards, squirrels, and a small host of other animals. They all glide beautifully, but none of them beats its gliding appendages in order to improve its flight characteristics. Empirically, therefore, we don’t seem to have any examples of gliders which are evidently along an evolutionary path towards flight, though gliding and parachuting are often perfectly effective adaptations in their own right.
But they look like terminal, rather than intermediate, states.
Exactly.
Does your research therefore support a cursorial model of evolution?
I would argue that there are Achilles’ heels in each of those models. There’s a problem with the cursorial theory: how do you explain an animal with massive legs and tiny wings using its wings to go faster or to pull itself up into the air? We don’t see that either in the world around us, which parallels the empirical difficulties of the arboreal theory. Are we seeing any intermediate adaptive stages that make sense to us? No. If the same pressures that drove animals as they were evolving flight are still driving them today, then we should be able to see remnants of, if not the exact traces of, animals striving to do today the same things that they needed to do in the Mesozoic. I would argue that studying the proto-wings of juvenile, still-developing birds might provide a useful avenue by which to approach the problem of the earliest flyers.
In our research, we’ve seen that partial wings give chicks a significant increase in performance, to escape or to find refuge, prior to their development of full-fledged flight. We’ve also seen how adults, even with the ability to fly, preferentially use wing-assisted running. Rather than concentrating on the arboreal/cursorial divide, I think it might be more fruitful to focus on questions like these, such as how does the flight stroke itself develop, what structures make it possible, and what purposes can they serve at differing levels of efficiency.
I think we’ll get closest to the truth when we use living organisms to show us processes, not just strict behavior, but morphological trajectories, and how various behavioral tendencies might have first developed in the living creatures of the past. I think we need to bring ecology, behavior, and developmental biology into the discussion of the evolution of animal forms and locomotion. This research is an example of how that might happen.
Dial’s videos of his WAIR experiments are available at the Flight Lab’s website: biology.dbs.umt.edu/flightlab/ [link defunct—Eds.].
Kenneth Dial is a professor in the Division of Biological Sciences at the University of Montana.
Jay Worthington is a lawyer based in New York City. He is an editor at Cabinet and the co-founder of Clubbed Thumb.