The Beautiful Possibility
Here comes the sun
Paul Collins
Back in the 70s, readers of Popular Science magazine were regularly treated to a parade of electrical gadgets, outlandish vehicles, and the latest discoveries in materials science, all promising to change our lives in the near, if slightly indefinite, future. One typical article carried this description of a contraption erected in France:
The traveler who visits the library of Tours sees in the courtyard in front a strange-looking apparatus. Imagine an immense truncated cone, a mammoth lampshade, with its concavity directed skyward... On the small base of the truncated cone rests a copper cylinder, blackened on the outside, its vertical axis being identical with that of the cone.
Thanks to solar boilers like this one, readers were assured, “future generations, after the coal-mines have been exhausted, will have recourse to the sun for heat and energy. ... The sun will be the fuel of the future.”
It is not strange that such an article was written in the 70s, when speculations on alternative power and the limited supply of fossil fuels filled Popular Science. But this issue is an unusual one; there are no references to the building of the Space Shuttle or to the Carter Administration, and no ads for Heathkits or for Tarryton smokers who’d rather fight than switch. In fact, there are no color graphics or photos at all. The issue is dated September 1876.[1]
“It has been a favorite pastime,” said Harper’s Weekly in 1903, “for the dreary gentlemen who juggle with statistics solemnly to calculate the date on which we shall all freeze to death from exhaustion of the coal supply.” The question was an old one; as early as 1829, a parade of geological experts were called to the House of Commons to give their estimates of when England’s coal fields would be mined out. Their guesses varied wildly, from as long as 1,700 years to as few as 200. But few could have predicted how coal usage would skyrocket, as industrial growth and the wiring of millions of new single-family homes for electricity made people giddy with the desire for more power.
By the turn of the century, it seemed that everything could and should be electrified. In 1909, the inventor George Knap designed and built an “Electrical Household” in the heart of Paris, featuring an elevator that delivered plates and tureens up to the diners, and a revolving platform to deliver dishes to everyone at the table, all controlled by push-buttons at the host’s chair. The kitchen had an electric range, electric dishwasher, an electric churn, an electric meat chopper, even an electric milk dispenser. Bedrooms were equipped with personal freight elevators, so that the morning paper might be sent up wordlessly to inhabitants. Knap’s house, explained Scientific American, even had “‘electric spies’ distributed in all rooms behind the wall paper,” for the benefit of the master of the household. A few years later Knap announced plans for an electric hotel in Paris, where a labyrinthine system of periscopes, dictographs, personal elevators, and revolving tables would insure that patrons would never have to lift a finger for anything.[2]
In the meantime Nikolai Tesla, who pioneered the AC power now used in every household, also considered plans for electrifying the dirt beneath our feet so that appliances wouldn’t need to be plugged in anymore. He helpfully suggested electrifying the floors of schoolrooms to keep students more alert. Later on, he proposed electrifying the planet’s upper atmosphere so that it would fluoresce like gas in a tube; it would be the end of night, a permanent office lighting for a future laboring beneath a buzzing celestial drop-ceiling.
But his favorite invention was rather more humble: the radiometer. It looks a little like a black windmill inside a glass bulb, and it spins when the sunlight falls upon it. It was, Tesla said, “the most beautiful invention” ever made.
• • •
Perhaps Tesla felt a powerful symbol in the laboratory toy, for it was solar energy, via coal and oil, that was powering this electrical frenzy. Direct solar power, though, has a long lineage. Archimedes used mirrors to set fire to Roman ships invading Syracuse in 212 B.C., though for the better part of two millennia this story was given little thought or credibility. But in 1747 George-Louis Leclerc, Comte de Buffon, arranged 168 individual mirrors to create a parabolic “burning mirror” and proceeded to set a pile of tarred planks afire from 195 feet away. Others had quietly experimented before Buffon, including Leonardo DaVinci, whose secret construction of a giant concave mirror and solar boiler was left unfinished at his death in 1519.
It was not until the late 19th century that a concerted effort was made to harness the sun’s power. The man behind the Tours library boiler, Augustin Mouchot, was a mathematics professor at the Lycée de Tours. When he began experimenting with solar energy in 1860, it was not with a parabolic mirror but with a little box. “Hot boxes”—imagine a miniature heat-trapping greenhouse ceiling built over walls and floors made of insulating black cork—had been pioneered by Horace de Saussure a century earlier. Astronomer John Hershel was inspired in the 1830s to hold little family cookouts with just such a box. “A very respectable stew of meat was prepared,” he reported, “and eaten with no small relish by entertained bystanders.”
Mouchot’s concerns went deeper than the stewpot. “Eventually industry will no longer find in Europe the resources to satisfy its prodigious expansion,” he warned. “Coal will undoubtedly be used up. What will industry do then?” The key was to develop enough solar energy to power a steam engine. Mouchot’s first crude hot box design, though useful for cooking, was not an economical way to create large quantities of heat for a boiler. You could, however, get intense heat from a parabolic reflector.
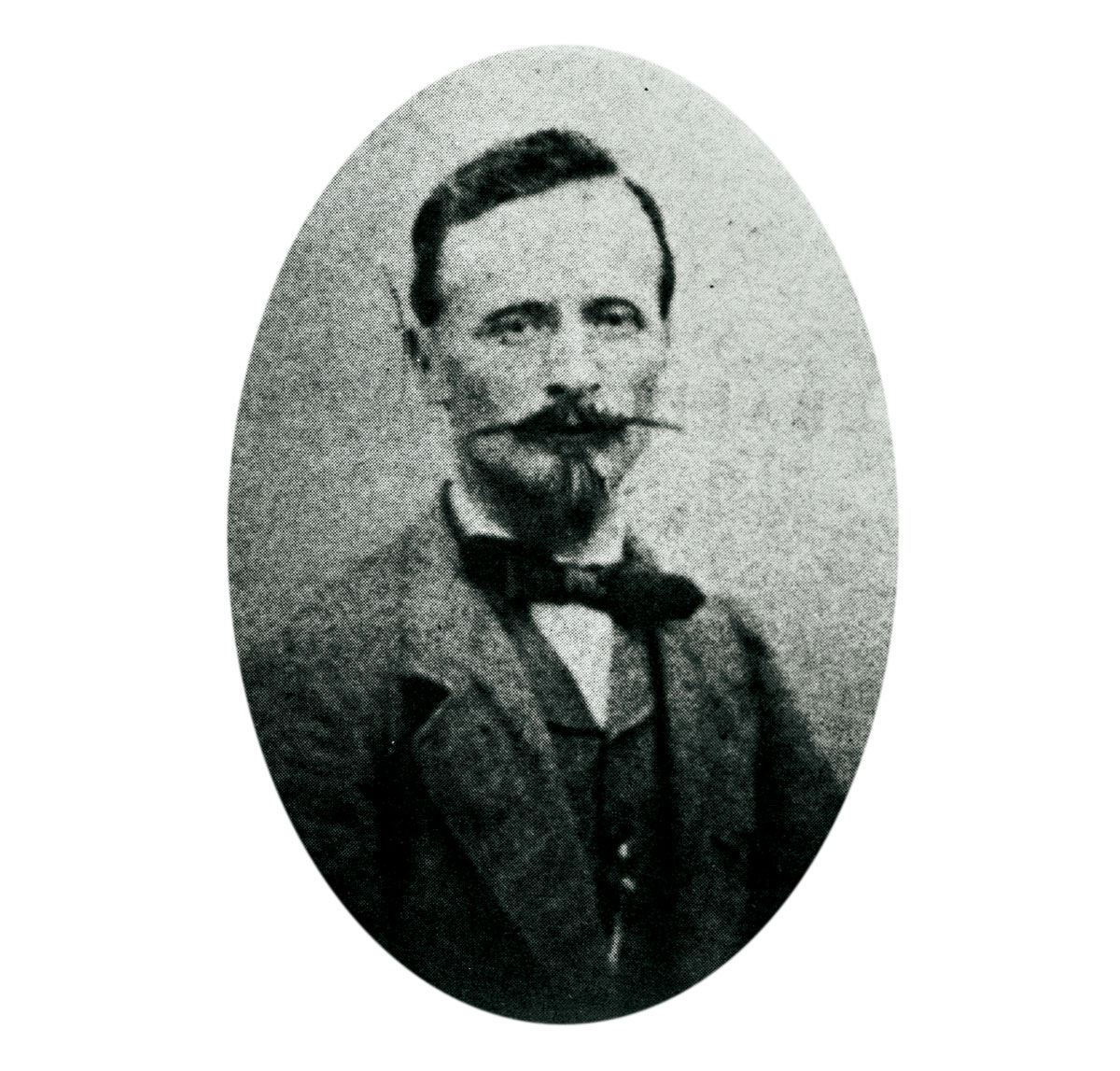
One of Mouchot’s countrymen was thinking of burning mirrors on a grand scale indeed. Charles Cros spent the same decade pondering how mirrors might be used to communicate with Martians. His solution, outlined in his 1869 book Moyens de Communications avec les Planètes, was bizarrely ingenious: Build a gigantic movable mirror with an extremely shallow parabolic curvature, for the more shallow the angle, the further away your focal point is. Make your focal point the surface of Mars, and the intense heat of the ray would fuse the Martian desert sand into glass. By moving the earth mirror you could, as it were, carve a greeting into a neighboring planet—a geometric shape, perhaps, as mathematics was the universal language. How else Martians might interpret a heat ray incinerating their land did not seem to occur to Cros.
Mouchot’s chances of success initially seemed little better than Cros’s. Mirrors could generate intense heat, but most of this energy was then lost. The trick was to generate the heat and conserve it: the mirror was very good at the former, and the hot box at the latter. And so in 1861 Mouchot received a patent for the Marmite Solaire, a burning mirror that focused on a heat-trapping glass jar. It could melt tin, lead, and zinc in a matter of minutes. With slight modification, solar distillers could make brandy in it and, the morning after, use it to brew themselves a much-needed pot of coffee.
Mouchot demonstrated his first solar engine in Paris in August 1866 to Emperor Napoleon III, and the inventor was funded for a more ambitious phase of building. Over the next few years, Mouchot built larger and larger machines, and poured himself into writing the first book ever devoted to solar energy: Le Chaleur Solaire et les Applications Industrielles (1869). Its publication coincided with the unveiling of his greatest engine yet. He built a sprawling mirror beneath a seven-foot copper boiler, a setup that generated a respectable 45 psi of steam. The monster engine was proudly displayed in Paris until the Prussian army seized the city in 1871, whereupon it disappeared.
Mouchot could not afford to rebuild his engine or continue his experiments for several years. When his chance finally came in the form of 1,500 francs from his local government, he quickly assembled the engine installed at the Tours library. In one test, its pressure rocketed so high that the boiler threatened to burst. The uses for this much power were quickly apparent to readers of Revue des Deux Mondes:
The aeronaut can with its aid propel his air-ship. Hot air motors and ammonia engines will be benefited by use of the solar receiver; but it is especially in tropical countries that it is destined to find immediate employment, in driving the various kinds of machinery used in sugar and cotton plantations, in crystallizing saline and saccharine solutions, in pumping water for irrigation, in manufacturing ice, etc.
The government was certainly impressed, for it employed Mouchot to build solar-powered projects in its possessions in Algeria, including some desalination plants that were still in use many years later.
Upon his return to France, Mouchot and his assistant, Abel Pifre, astonished audiences at the 1878 Paris Exhibition. Mouchot’s exhibition engine was weighty, with a mirror over 13 feet in diameter and a 21-gallon boiler. One sunny day out on the Trocadéro, Mouchot generated seven atmospheres of pressure in his boiler, set up an ice-maker, and produced a solar block of ice. Bystanders puzzled over the paradox of a running a furnace to create ice, and it so tickled the judges’ fancy that Mouchot was awarded a Gold Medal.
But the greatest stroke of showmanship was by his protégé, Pifre. Mouchot had published a farewell edition of Le Chaleur Solaire in 1879, giving up solar research to return to teaching. Pifre immediately built several more motors and then, erecting one in the Tuileries, hooked it up to a printing press, creating sheet after sheet of Le Journal du Soleil, a newspaper that he had created especially for the event. The press rattled off 500 copies an hour, which Parisian strollers read, marveled at, and then, in all likelihood, discarded.[3]
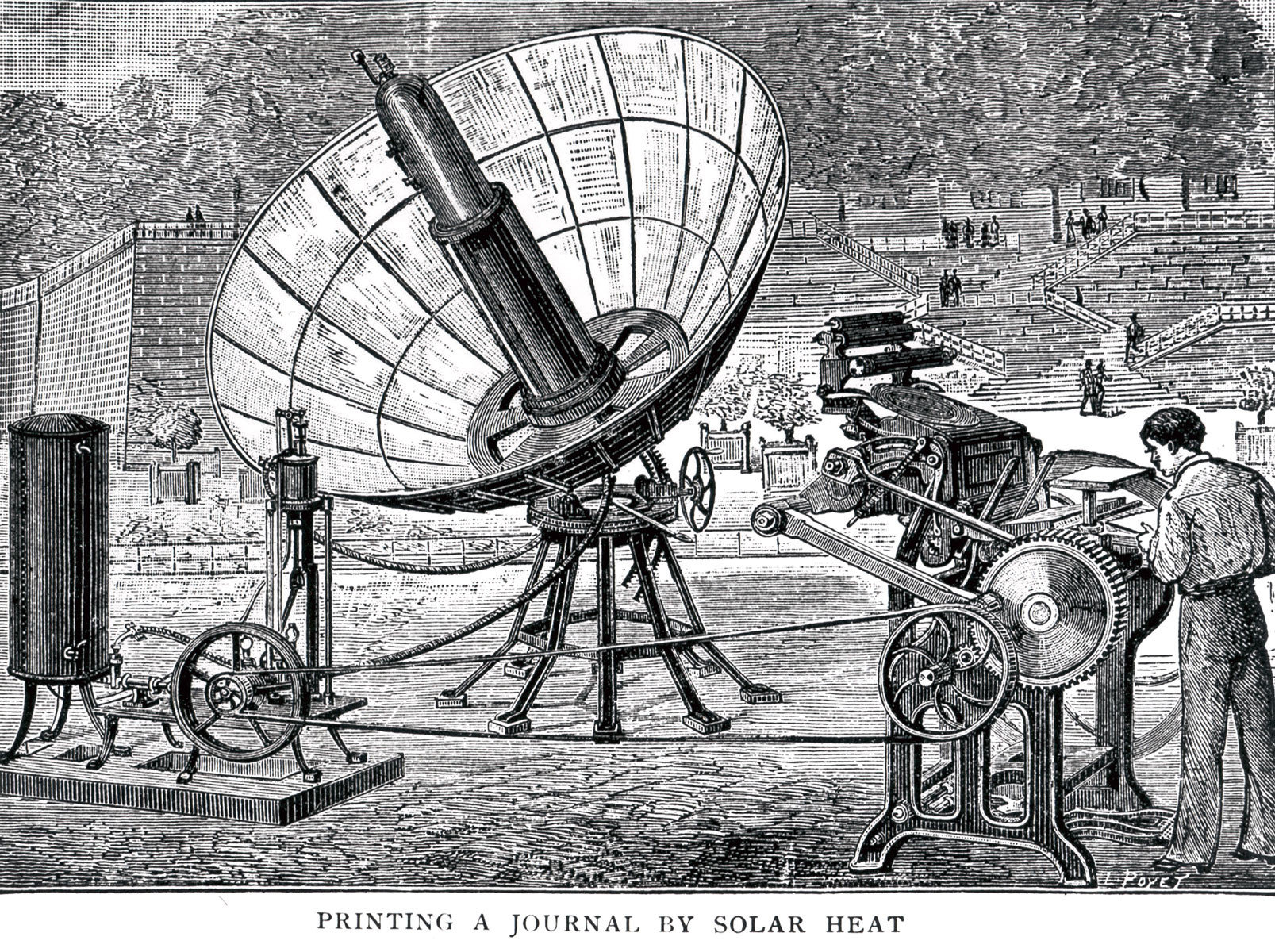
“A mere toy,” was John Ericsson’s judgment of the French engine—though the advisability of giving children a 2,000-degree furnace to play with is open to question. His criticism of Mouchot was rude, but Ericsson was a man supremely confident in his own talents: “Archimedes, having completed his calculations of the force of a lever, said that he could move the Earth,” he wrote. “I affirm that the concentration of the heat radiated by the sun would produce a force capable of stopping the earth in its course.”
It was not an idle boast. After Edison and Tesla, Ericsson was probably the greatest American engineer of his era. Born in Sweden in 1803, he already had thirty patents to his credit by the time he immigrated to America in 1839. He’d created furnaces, desalination machines, and a new type of replacement for the steam engine, the “hot-air engine.” He’d also invented the screw propeller for ships. Ericsson first came to prominence in America, though, with a revolutionary “caloric engine” that recovered the heat lost during operation. This engine, installed in the prosaically named paddlewheel boat Caloric Ship Ericsson, would only need an occasional small flame to keep it running after the initial firing-up. There was just one problem: his heat-traps didn’t actually work. The Laws of Thermodynamics—which essentially forbade his near-perpetual motion machine—were not well understood yet. Unfortunately for Ericsson, ignorance of The Laws is never an excuse, and the engine wouldn’t work, no matter how hard he pleaded with it.
All the same, by the 1850s Ericsson was perhaps the best-known engineer in the country. His reputation was made permanent when he designed and built the Monitor, the famous ironclad warship that, in a single instant in 1862, had rendered every navy in the world obsolete. Once the Civil War was over, Ericsson thought of what he might do for generations yet unknown. “A couple of thousand years dropped in the ocean of time will completely exhaust the coal fields of Europe,” he mused. The nautically inclined engineer spent much time pondering how to harness tidal power, but this proved a dead end. Solar power seemed a better bet; while it might not be appreciated now, in some future era it surely would be society’s savior. “The field awaiting the application of the solar engine is almost beyond computation, while the source of its power is boundless,” he said. “Who can foresee what influence an inexhaustible power will exercise on civilization?”
Ericsson decided to devote the rest of his life to it. Perhaps it was also in Ericsson’s nature to pursue that fondest pipe dream of every engineer: something for nothing. Unlimited and efficient solar power was not so different in its allure than his ill-fated caloric engine. He bought a house at 36 Beach Street in Manhattan, built a solar observatory on the roof and a wooden engine platform in the garden, and set about in earnest to create his first solar engine.
For all his mechanical genius, Ericsson could be curiously stubborn about some technologies. He refused to ride elevated trains or to believe that telephones actually worked, and he hated both modern plumbing and the newly invented typewriter; when a typewritten letter arrived, he would insist that it be copied into script before he’d read it. He refused throughout his old age to use reclining chairs: They were built, he insisted, upon false mechanical principles. And when rats plagued the Beach Street house, he spent days with most of his machine shop staff contriving a massive rat-trap until it filled half his basement. It was essentially a trap door over an elaborate chute and water tank, but the rats wouldn’t take the cheese.
Most of the time the engineer could be found in his office, working on his next bigger and better engine, as his friend William Church recalled:
He sat at his work upon an ordinary horse-hair piano stool raised to a convenient height by the addition of a rough wooden box, unpainted, and polished only by use. This box, or a dictionary, served him for a pillow when he turned aside from his work to stretch himself out at full length for a nap on the table standing opposite his desk. Until the bright idea of lengthening it occurred to him one day, he slept most uncomfortably with his legs dangling over the edge of the table.[4]
Ericsson spent the 1870s developing sun-motors, and adopted silvered glass for his mirrors, as it was cheaper, easier to replace, and easier to clean. This, along with his superior fabrication skills, meant that his engines had a far higher output and smaller size than Mouchot’s. The engineer sensed victory; as one engine neared completion, his final judgment of it to a friend was simple and confident: “It marks an era in the world’s mechanical history.”
• • •
There was one intractable problem: The sun sets. In the 18th century, the inventor Horace De Saussure had bravely attempted to generate heat from moonlight, but failed. Now, a century later, Ericsson was equally confounded by the problem of generating solar power at night. Batteries were hardly advanced enough yet, so the most promising route was raising water in a tower during the day, and releasing its potential energy at night by dropping it through a dynamo. Unfortunately, none of these approaches resulted in a truly satisfactory and economically competitive product.[5]
As ruthless a critic as he could be on competitors like Mouchot, Ericsson had even less patience for his own mistakes, and by 1880 the engineer’s designs had undergone radical alterations. He dispensed with steam altogether as a means of running an engine, instead focusing on the Hot Air Engine, which could be operated at lower temperatures and with greater safety and ease than steam. To his chagrin, he could never get it to run as efficiently on solar power as he would have liked. Delameter & Company sold over 50,000 of his hot-air engines in the 1880s—“Any Servant Girl Can Operate It!” boasted their ads—but they were almost all fueled by wood, coal, or gas.
Undaunted, Ericsson built more sun-motors throughout the 1880s, growing more confident of the eventual utility of his invention. “You will probably be surprised when I say that the sun-motor is nearer perfection than the steam-engine,” he wrote one friend, “but until coal mines are exhausted its value will not be fully acknowledged.” He calculated that solar power cost about ten times as much as coal, so that until coal began to run out, solar power would not be economically feasible. But this, to him, was not a sign of failure—there was no question that fossil fuels would indeed run out someday.
The great engineer maintained an unshakeable belief in the future of solar power to his last breath; he had set up a large engine in his backyard and was still perfecting it when he collapsed in early 1889. Though his doctor made him rest, Ericsson could not sleep at night: he complained that he could not stop thinking about his work yet to be done. As the journal Science marveled after his death a few weeks later:
As he saw the end approaching, he expressed regret only because he could not live to give this completed [solar] invention to the world in its completed form. It occupied his thoughts up to his last hour. While he could hardly speak above a whisper, he drew his chief engineer’s face close to his own, gave him final instructions for continuing the work on the machine, and exacted a promise that the work should go on.
In spite of his dying wishes, he did not make it easy for his colleagues to follow in his footsteps. When the Stockholm Museum asked him the year before his death for his records, they received this tart reply:
Accept my thanks, but permit me to inform you that I take no interest in museums which preserve relics of barbarism and ignorance of past times .... I have already destroyed upward of one thousand drawings, and numerous models, to prevent posterity from supposing that my knowledge was as imperfect as said relics would indicate. Nothing will be left at last but the corpse of
JOHN ERICSSON.
New York, June 19, 1888
We will never know how close he was to perfecting solar power. He was buried on 11 March 1889, with only empty torn-out bindings left on the shelf where he had kept his laboratory journals.
• • •
Solar engines based around intense burning mirrors may have always been doomed. Hindering every inventor were the mirrors themselves—they were expensive, heavy, dulled when exposed to the elements for long, and were vulnerable to wind damage. The logical response to this from an engineer might be: Why not get rid of the mirrors? Thus Charles Tellier, who had already invented modern refrigeration, looked very closely at creating low-temperature solar motors that would run off of blackened surfaces to heat up water or, better yet, lower boiling-point liquids like ammonia or sulfur dioxide.
The problem with low-temperature passive surfaces is that generating a significant amount of energy requires a lot of space for the expanses of collectors. This wasn’t practical in individual city dwellings. But the end of the century saw a switch to the alternating current pioneered by Nikolai Tesla. AC can be transmitted over long distances—the generators don’t have to be on valuable urban space. Put solar engines on cheap desert land, where the sun shines longer and hotter anyway, and you can sprawl out all you want. St. Louis inventors H. E. Willsie and John Boyle, Jr., grasped this, and beginning in 1904 their Willsie Sun Power Company built solar power plants in St. Louis and in the deserts of California and Arizona. Their Needles plant generated a respectable 11 kilowatts, showing that power could be generated by desert plants, and distributed on a scale more cheaply than Ericsson had ever imagined.
The question now was: Who was going to do it first?
Tacony is a town that is little heard of today, save for the car dealership ads that run in the Philadelphia Inquirer. Yet it held a dream once—one of such unimaginably grand proportions that it has hardly been equaled since. Frank Shuman was a local inventor in Tacony at the turn of the century, and he found himself drawn into tinkering with solar power. By 1907 his experiments filled his backyard, where he erected a 1,200-sq-ft collection area to power a water pump; during the summer, neighbors watched the three- horsepower contraption pumping thousands of barrels of water a day. But the engine was not perfect; for one thing, it initially cooled off so much overnight, and took so long before it begrudged any work the next day, that a reporter wondered aloud “if it belonged to the Sun union.”
Shuman eventually figured out how to prevent the heat loss, and like most other solar engineers, he generated power at night by using an insulated water tower. Within a couple of years of these backyard experiments he was issuing stock for The Sun Power Company and landing financing from a group of English investors. Along with this financing came two engineering partners, A. S. E. Ackerman, and Professor C. V. Boys. Shuman and his crew packed their bags in 1912 and set off for the ultimate destination of every solar engineer, one that had lain dormant for forty years since the last visit of Mouchot: the Sahara Desert.
Shuman’s vision brought him to Meadi, a farming town about 15 miles from Cairo, where he bought some land and hired cheap local labor to build the largest solar power plant the world had ever seen. When it was finished and opened in July 1913, it had over 13,000 square feet of collectors powering a 55-horsepower irrigation system. The Meadi plant ran 24 hours a day, just as it was designed to do, and it was built to last of simple but strong materials like concrete and steel.
One visitor to Meadi was Lord Horatio Kitchener, the Consul General of Egypt. Watching the powerful pumps operating by the ghostly burning power of the Egyptian sun, Kitchener couldn’t help but think that this might indeed be the future of power. He offered Shuman an entire 30,000-acre plantation in the Sudan to build his next irrigation system. Not to be outdone by its greatest rival, Germany called a special session of the Reichstag to host Shuman, an unprecedented event there for an inventor. They, too, now wanted solar plants in their African possessions, and dangled $200,000 before The Sun Power Company to build one for them.
Shuman was jubilant. Solar power, he boasted, was now inevitable: “There is not a single ‘should give’ or ‘guess’ about it. Sun power is now a fact, and is no longer in the ‘beautiful possibility’ stage. It can compete profitably with coal in the true tropics now.” So, he reasoned, why not spread the plants across all of Saharan Africa and generate electricity with them? With AC power being sent over longer and longer distances now, there was no reason that the Sahara could not power all of Europe. Shuman’s idea unfolded to readers in the 24 February 1914 issue of Scientific American:
For the purpose of my article I have taken as a basis the figure of two hundred seventy million horse-power continuously throughout the year being equal to all the coal and oil mined during the year 1909 throughout the world. ... Taking the actual work of our plant as a basis, it would only be necessary to cover 20,250 square miles of ground [i.e. a square of 143 miles] in the Sahara Desert with our sun heat absorber unit ... to give perpetually the two hundred and seventy million horse-power per year. ... Surely from this showing, the human race can see that solar power can take care of them for all time to come.
Now, the next question your reader will ask is, “How much will it cost to do all this?” Of course the figure will be staggering, being equal to ninety-eight and one-half billion dollars ... [But] we will then have a plant that is worth to us as least as much as all the coal and oil fields in the whole world; because it can perpetually give as much heat and power as all of the coal fields and oil fields of the world put together, if mined at the 1909 rate. And these are certainly worth very much more than ninety-eight odd billion dollars.
This vast investment would not be made for or by the individual, but for and by the entire human race.
Perpetual power, clean and guaranteed, and financed, Shuman suggested, by spreading out the cost over a long period of time with bond issues. All it needed was the will to build it. Like most solar engineers, Shuman was alert to the political and environmental havoc that fossil fuels might wreak upon the world. “One thing I feel sure of,” Shuman insisted, “and that is that the human race must utilize direct sun power or revert to barbarism.”[6]
But as the events of 1914 were to prove, humanity was to revert to barbarism much sooner than even Shuman imagined.
Neither Shuman nor his power plant survived to see the end of The Great War; in the shattered aftermath, spending $98 billion on anything became simply unthinkable. It is hard to realize today that before the war, the struggle for energy primacy had not yet quite been won. For a time, there were more horseless carriages running on steam and electricity than on gasoline; predicting which power source would triumph was a dicey proposition. But war has a selective effect on technology. Gas and oil engines—cheap and powerful, always important factors in a war—improved very rapidly. By the war’s end, fossil fuel technology and Anglo-American petroleum holdings had begun to establish a dominance that would determine international politics and effectively choke off alternate fuels. Solar power has remained the preserve of idealists, a backwater to a sea of oil, gas, and coal, doomed to be discovered and forgotten and then rediscovered over and over again. It is a little galling to think that nearly a century ago, when Shuman’s gleaming panels faced the same sky that darkens over us today, they looked so much like the future.
They still do.
Further Reading
The best starting points are Mouchot’s Le Chaleur Solaire et Ses Applications Industrielles (1869, 1879), Charles Henry Pope’s Solar Heat: Its Practical Applications (1903), and Ken Butti and John Perlin’s A Golden Thread: 2500 Years of Solar Architecture and Technology (1980).
- See Popular Science Monthly (September 1876, April 1877); the former is a translation of a 1 May 1876 article in Revue des Deux Mondes.
- See Scientific American for 4 December 1909 and 1 November 1913.
- This newspaper has been variously identified as Soleil Journal, Le Journal Soleil, and Le Journal du Soleil. Neither the Library of Congress, the British Library, nor the Bibliothèque Nationale de France lists any of these titles, though the latter does have numerous other works by Mouchot and Pifre. Also see the 13 May 1913 issue of Scientific American, and volumes 59, 81, 86, and 91 of Comptes Rendus.
- William Conant Church, The Life of John Ericsson, Volume II (London: Sampson Low, Marston and Company, 1892), p. 314.
- De Saussure’s moonlight idea is described in Every Saturday, 18 September 1869.
- Also see Scientific American for 21 January 1911, 1 November 1913, and the Engineering News for 13 May 1909.
Paul Collins lives in Portland, Oregon. He is the author of Banvard’s Folly: Thirteen Tales of Renowned Obscurity, Famous Anonymity, and Rotten Luck (Picador, 2001), and edits the Collins Library for McSweeney’s Books.